As Moalem takes readers through the different factors that can affect evolution and the human genome, it appears at first that the cliché phrase “biology is destiny” might be true, as various evolutionary pressures select for advantageous genes. Yet in the later chapters of the book, Moalem makes a point of illustrating the ways in which DNA can adapt at early stages of an individual’s life. Even though one’s genetic information might be inherited from one’s parents, Moalem illustrates how several biological processes can enable the expression of seemingly new traits and allow us to adapt at a much more rapid pace.
Epigenetics is an emerging field that studies how the resources a fetus receives when inside its mother’s womb, and in the first few months after a child is born, can significantly affect how traits are expressed in a child. This suggests that even if one’s genes are not altered, how those genes are expressed might not necessarily be set in stone before (or even after) birth. The first big breakthrough in the field of epigenetics came from a study of agouti mice, which are distinct for their yellow coats and a tendency toward obesity. A team of scientists at Duke University then separated pregnant agouti mice into two groups. In the control group, they gave the mice a regular diet, and their babies were also fat and yellow. In the experimental group, they fed the pregnant mice vitamin supplements of vitamin B12, folic acid, betaine, and choline. Their babies, by contrast, were thin and had a dark coat. This suggested that certain compounds in the vitamin supplements were able to “turn off” parts of their genes. Their DNA still contained the gene for pale coats and fatter bodies, but the chemicals had “attached to the gene and suppressed its instructions,” a process called methylation. The results of this experiment thus suggest that even if genes are fixed, the expression of those genes is not. The same kinds of results have long been found in other animals in the wild, where researchers observed how the offspring of some organisms can be “custom-tailored on the basis of the mother’s experiences during pregnancy.” For instance, the mother of the tiny freshwater flea Daphnia will produce offspring with a larger helmet and spines if it’s going to give birth in an environment crowded with predators. Additionally, one species of lizard is either born with a long tail and large body or a small tail and small body depending on whether its mother smelled a lizard-eating snake while pregnant. These examples serve to show how the environment and resources of a mother can greatly impact her direct offspring. These adaptations are vastly different from genetic mutations that have developed and been handed down over time; instead, these examples prove how some adaptations can develop in a single generation. Methylation is present in humans as well, and it’s one of the reasons why child obesity may have become more prevalent in recent decades. If a newly-pregnant mother eats junk food that’s largely devoid of nutrients, “the embryo may receive signals that it’s going to be born into a harsh environment where critical types of food are scarce.” In response, various genes are turned off through methylation, and the baby is born small and with a “conservative metabolism” so that it needs less food to survive. Then, when the child grows up in a nutrient-poor but calorie-rich diet, the child gains weight. This illustrates the impact of prenatal nutrition on children throughout their lives: even if they do not inherit obesity, children can acquire traits before birth that lead to this condition.
Methylation is not the only way in which our genes can rapidly adapt. The discovery of “jumping genes” proves that even our genetic material can change in order to direct the process of adaptation, particularly under times of stress. Barbara McClintock studied genetic mutation in corn in the 1950s. Moalem describes how “Especially when the plants were stressed, McClintock discovered whole sequences of DNA moving from one place to another, even inserting themselves into active genes. When these genes cut and pasted themselves from one place in the corn’s DNA to another, they actually affected nearby genes—by changing the sequence of DNA, they sometimes turned genes on and sometimes turned them off.” Moalem summarizes that the corn plants seemed to be engaged in “intentional mutation,” thereby uncovering another method by which organisms can alter their genetic blueprint. These “jumping genes” are called transposons; in addition to corn, they’ve been found in organisms like fruit flies, the bacterium E. coli, and even humans. For us, jumping genes are “are very active in the early stages of brain development […] Every time one of those jumpers inserts or changes genetic material in brain cells, it's technically a mutation. And all of that genetic jumping around may have a very important purpose—it may help to create the variety and individuality that make every brain unique.” Thus, jumping genes not only help us change our genetic code, but they may also allow for our individuality as a whole. Jumping genes also enable us to create antibodies—specialized proteins to target specific invaders. This idea makes a particularly strong case for how we may have adapted jumping genes, as these mutations directly lead to our enhanced survival in fighting viruses and bacteria.
Research on epigenetics and jumping genes is still in the early stages—it’s not entirely clear what spurs either process or exactly how different stimuli affect mutations and genes. Moalem even writes a note of caution that “we need to be awfully careful when we start to change the choreography”—that is, the choreography of how the building blocks of our genetic code are formed. But these two new avenues of research clearly illustrate that our biological code is not set in stone, and that these adaptations allow for a vast amount of future evolutionary possibility.
Genetic Expression, Acquired Traits, and Mutation ThemeTracker
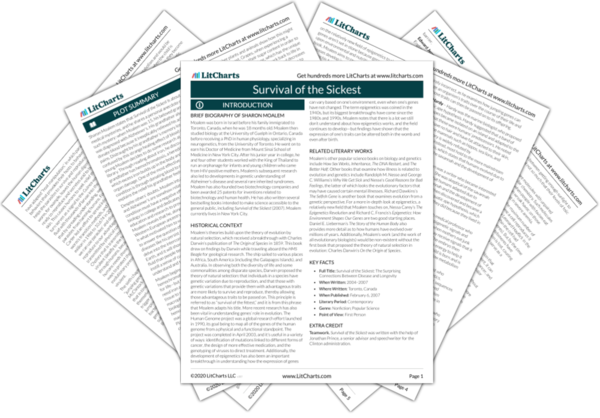
Genetic Expression, Acquired Traits, and Mutation Quotes in Survival of the Sickest
By introducing the harmless virus to our bodies, we stimulate our immune systems to produce antibodies specifically tailored to defend against that virus. Then, if we are exposed to the harmful version, our bodies are prepared to defend themselves immediately.
McClintock believed that the jumps are a genomic response to internal or environmental stress that cells can't handle under their existing setup. Essentially, a challenge to survival triggers the organism to throw the mutation dice, hoping it will land on a change that will help. That’s what she thought was going on with the corn plants she was studying—too much heat or too little water triggered the corn to gamble its survival on finding a mutation that could help it survive.
According to Villarreal, this capacity of African primates to support the persistent infection of other viruses may have put our evolution on “fast forward” by allowing more rapid mutation through exposure to other retroviruses. It’s possible that this capacity helped spur our evolution into humans.
Essentially, one or more of the compounds in the vitamin supplements fed to the expectant mothers reached down into the mouse embryos and flicked the agouti gene into the “off” position. When the baby mice were born, their DNA still contained the agouti gene, but it wasn’t expressed—chemicals had attached to the gene and suppressed its instructions.
According to the thrifty phenotype hypothesis, fetuses that experience poor nutrition develop “thrifty” metabolisms that are much more efficient at hoarding energy. When a baby with a thrifty phenotype was born 10,000 years ago during a time of relative famine, its conservationist metabolism helped it survive. When a baby with a thrifty metabolism is born in the twenty-first century surrounded by abundant food (that is also often nutritionally poor but calorie rich), it gets fat.
Here’s the first thing we don’t know—we don’t have anywhere near a complete understanding of which genes are turned off or turned down by which methyl donors. For example, methylation of a gene that influences hair color might lead to a harmless change—but the same process that triggered methylation of the hair color gene may also be suppressing a tumor suppressor.
I hope that you’ll come away from this book with an appreciation of three things. First, that life is in a constant state of creation. Evolution isn’t over—it’s all around you, changing as we go. Second, that nothing in our world exists in isolation. We—meaning humans and animals and plants and microbes and everything else—are all evolving together. And third, that our relationship with disease is often much more complex than we may have previously realized.